Some 5 years ago, imec began work on a unique chip that could sort single cells in a microfluidic channel. The technology holds the promise to one day revolutionize the way we diagnose and treat diseases.
A recent study focused on its use in cell therapy manufacturing. In this study, it was demonstrated that the cell-sorter chip is capable to sort out T-cell populations while preserving viability and potency. By adding cell focusing and optical waveguides to the design, progress was made towards a true multi-marker multichannel platform. This will enable very precise and high-throughput cell sorting for safer and more effective cell therapies for cancer treatment.
Liesbet Lagae, program director Life Science Technologies at imec, and colleague Sarah Libbrecht, senior bio-application specialist at imec, explain the new results achieved with the cell sorting chip.
How does the cell sorter chip work?
In 2014, in the framework of a prestigious ERC project (SCALPEL), Liesbet Lagae and her colleagues started work on imec’s cell sorter chip. Liesbet Lagae: “This chip of only a few cm² in size, is able to sort specific cells out of e.g. a blood sample by pushing and sorting individual cells into a microfluidic channel using a principle that is borrowed from inkjet print heads. In numbers: it can sort 5,000 cells/sec per single microfluidic channel, with a > 90% cell sorting yield, up to 99% purity and well-preserved cell viability. The cell sorter output is compatible with single-cell sequencing workflows and could in the future become an indispensable tool for more precise cancer diagnosis, for follow up and to monitor the efficacy of recently introduced, very expensive immune therapies.
This video describes its unique working principle. Basically, it uses micro vapor bubbles (created by micro-heaters) generating a jet flow for high speed, but gentle, cell sorting in a microfluidic channel.
Can the cell sorter chip improve therapeutic efficacy of CAR-T cell therapy and bring it to clinical applications?
In 2018, as a result of the initial technology developments in SCALPEL, Liesbet Lagae embarked on a second project (JetCell), also funded by the ERC. In this second project, the aim was to find out if the same technology could be used to improve cell therapy.
Cell-based cancer therapy is one of the most promising new cancer treatments. In particular, CAR-T cell therapies are getting popular. It is based on the patient’s own immune system (T cells) which is boosted to fight cancer. Regularly, new clinical approved therapies are reaching the market, and hundreds of start-ups are launched in the cell therapy field. Last month, GrandView Research projected a more than doubling of the global cancer immunotherapy market, from the $58.1 billion it tallied in 2018, to $126.9 billion by 2026 (source: GEN).
Liesbet Lagae: “In a typical T cell therapy workflow, relevant T cells are isolated from the patient blood, modified to recognize certain cancer markers, infused back to the patient body where the engineered T cells direct the immune system to attack and finally eliminate the malignant tumor cells and tissues.”
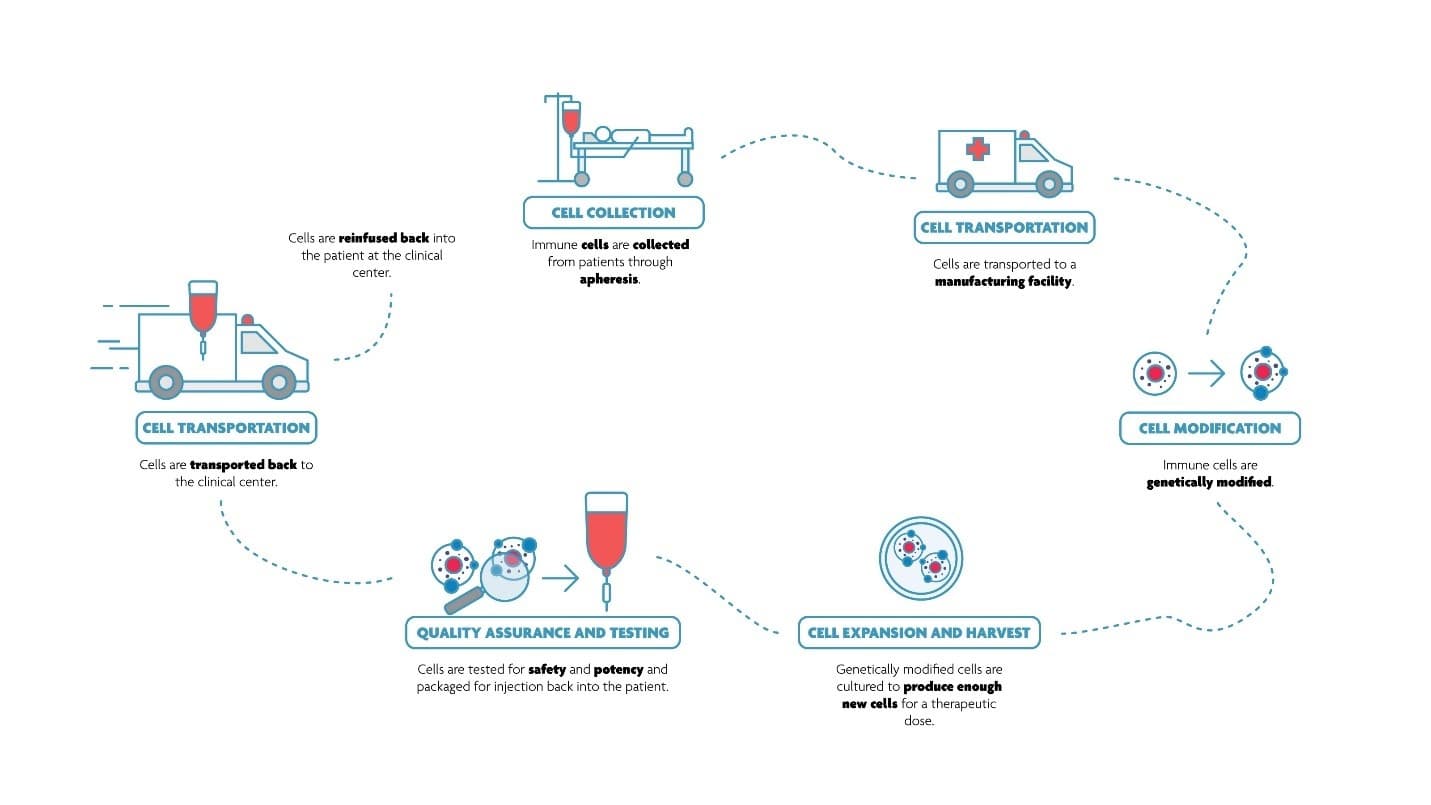
A typical process flow for cell therapy.
From R&D to clinical application. At several steps of the manufacturing flow (patient cell isolation, cell modification, cell expansion, quality assurance), therapeutic T cells need to be selected out of a pool of cells and other compounds. Current techniques include fluorescence activated cell sorting (FACS) and magnetic bead-based assays (MACS).
Liesbet Lagae: “From our discussions with cell therapy product developers, we learned that they are generally happy with the flexibility and performance of MACS and FACS as a cell separation tool for R&D applications. However, when cell therapy application will be ramped up, these experts see serious issues to use these conventional tools for clinical applications because of its complexity in operation, lack of automation, risk of contamination and carry-over (due to generation of aerosols), and time constraints in logistics, sample and tool preparation. In short, those research flows are not compatible with Good Manufacturing Practices (GMP) which form the basis of every pharmaceutical production flow.
High-throughput system. To become commercially viable and ensure that the patient gets his/her treatment in a reasonable timeframe, it is important that the cell sorter technique used is not only very precise but also very high throughput. Liesbet Lagae: “Our microfluidic chip is based on semiconductor technology, the champion in integration and mass-production. The tiny structures of an individual cell sorter can be produced in hundred and thousand-fold on one integrated chip, all working in parallel to purify a batch.”
Precision is key. Next to this, cell therapy manufacturing requires a very precise cell sorter tool. This would be a tool that can select out cells based on multiple markers. Today, even for approved batches (requiring a lot of manual tests), the efficacy of the therapy is not always high. This is probably because the cells are more heterogeneous than originally thought. Liesbet Lagae: “Current magnetic bead-based assays are not single cell and are not easily portable to multi-marker assays. With our cell sorter technique, every single cell in a batch could be screened and sorted, and multiple markers can be used for this selection process. The technology could even discriminate between high and low expression levels of membrane proteins/markers.”
GMP in a box. The big footprint and high cost of current tools do not favor its use in decentralized settings. Indeed, the concept of GMP-in-a-box where the whole process of cell manufacturing is implemented in a closed box system requires critical components to be compact and cost efficient.
Also, for cell therapy, FDA and GMP guidelines explicitly recommend closed systems to ensure the safety of the patients. A closed cartridge automated system, disposable (one for each patient) and without hands-on operations, is the preferred future use scenario that the cell therapy community is striving for. It is critical for the long-term success of autologous therapies such as the CAR-T cell therapy for cancer. Liesbet Lagae: “With microfluidic chips such as our cell sorter chip, such use scenarios are coming into reach. Of course, this would require a full system development: a microfluidic chip, instrument hardware and software.”
Demonstrating the potential of microfluidic ‘jet’cell sorting for T cell selection
A first important question to answer was if the cell-sorter technology is suitable for selecting T-cells.
Sarah Libbrecht: “There are two things that are essential for T cell manufacturing: purity and viability. As a proof of concept, we aimed to purify T helper cells, identified as CD4+ and CD14-. In standard practice, this cell population is isolated using antibody-labeled magnetic beads, often in a two-step isolation procedure. Using the cellsorter device, this isolation can be performed in a single step using fluorescently labeled antibodies.”
“We obtained a purity of 86%. Moreover, there is still room for further optimization in the selection criteria used to further increase the purity in order to meet the demands of clinical manufacturing.”
“In order to assess the viability of the sorted cells, we monitored the growth and expansion according to standard manufacturing procedures. As expected, our gentle method of cell sorting results in viable cells that are able to grow and expand in a similar way to MACS isolated cells.”
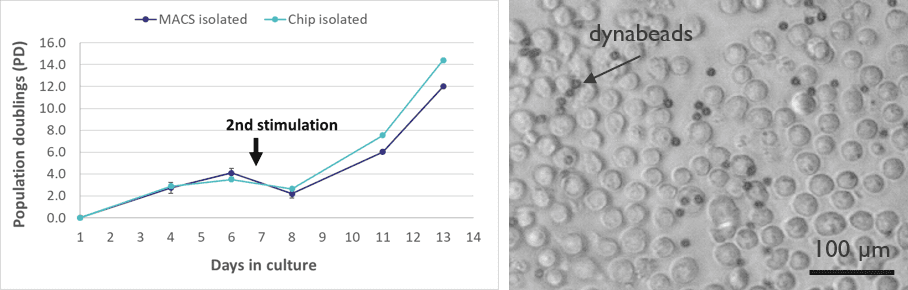
Growth curve (left) of T helper cells after isolation-on-chip versus the standard isolation using magnetic beads. T cells are observed healthy and interactive with the dynabead expansion beads (right).
Demonstrating the high-throughput potential of the cell sorter for T cell selection.
CarT-cell sorting applications demand a very high throughput cell sorting, up to a billion cells. This is very challenging for single channel sorting due to the high cell shear rate and the consequential damage to cell viability at this extremely high flow speed. A straightforward approach for high-throughput cell sorting is parallelizing sorting in multiple channels. This is particularly feasible with microfluidic cell sorting because of the inherent technology scalability. This is a key technology differentiator from other competing technologies such as commercial FACS and magnetic bead isolation.
Sarah Libbrecht: “In a first design, independent inlet/outlet holes and connection channels were used for each sorting channel to ensure hydrodynamic focusing of the cells at the detection spot. However, this will be too complex and space-demanding when hundreds and thousands of channels are used. Using this proof of concept, we were able to demonstrate that acoustic cell focusing can be a promising approach when going to tens, hundreds or even thousands of channels. Compared to other focusing techniques, acoustophoresis is a relatively inexpensive but effective method. Moreover, acoustophoresis is biologically friendly and gentle for cells, which is suitable for a continuous high-throughput manipulation of cells in microfluidics.”
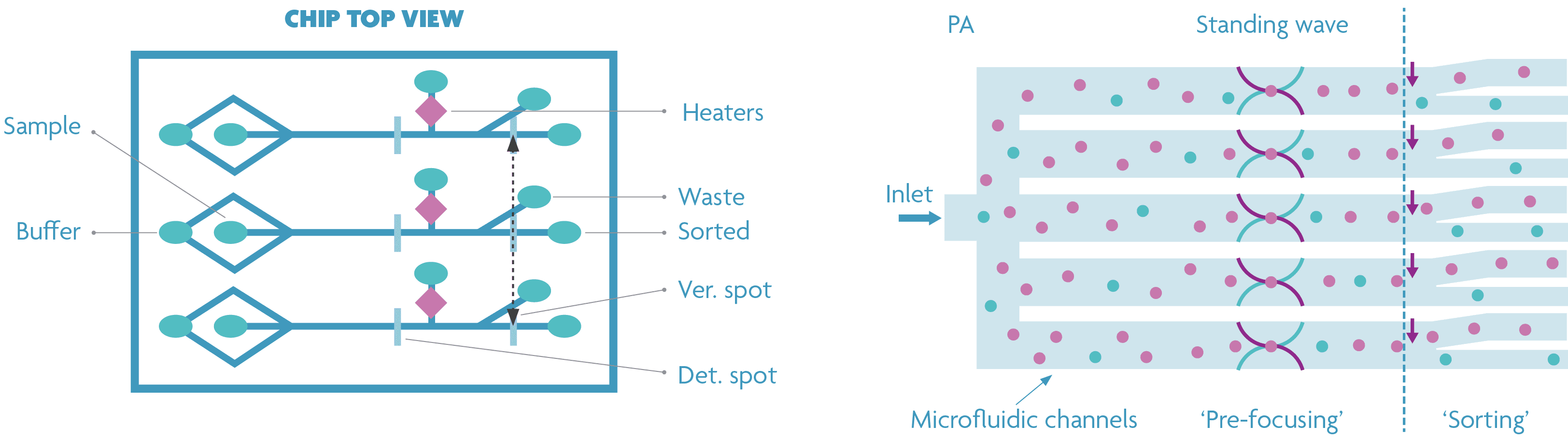
Standard (left) and optimized (right) design demonstrating the cell sorter’s potential for parallelization. In the optimized design, acoustic cell focusing is used by gluing a piezoelectric material to the backside of the chip.
Sarah Libbrecht: “Results showed that the acoustic cell flow focusing did not influence the bubble jet sorting (e.g. the creation and breakdown of micro bubbles) and vice versa. We will further improve on this principle and integrate it with the cell sorting to create a truly scalable multichannel sorting platform. Also, the optical system needs to be largely parallelized to allow independent cell detection per channel to realize truly independent multi-channel sorting.”
Demonstrating the high precision (=multi-marker) potential of the cell sorter
Multi-marker detection is key in making cell therapy safer and more effective. It does make the system optics rather complex, combining multiple channels and multiple markers. The latter corresponding to multiple wavelengths that individually need to be optically aligned and sensed simultaneously with real-time decisions to steer the cell sorter.
Sarah Libbrecht: “We are tackling this challenge by using on-chip photonic waveguides distributing the desired wavelengths for detection of different fluorescent markers to the microfuidic channels. We built an optical model considering the microfluidic channels, the waveguide excitation optics, the optical collection optics, and commercial detectors. Based on extensive simulations, we derived an architecture of cell sorter channels with integrated waveguides for multimarker / color detection.”
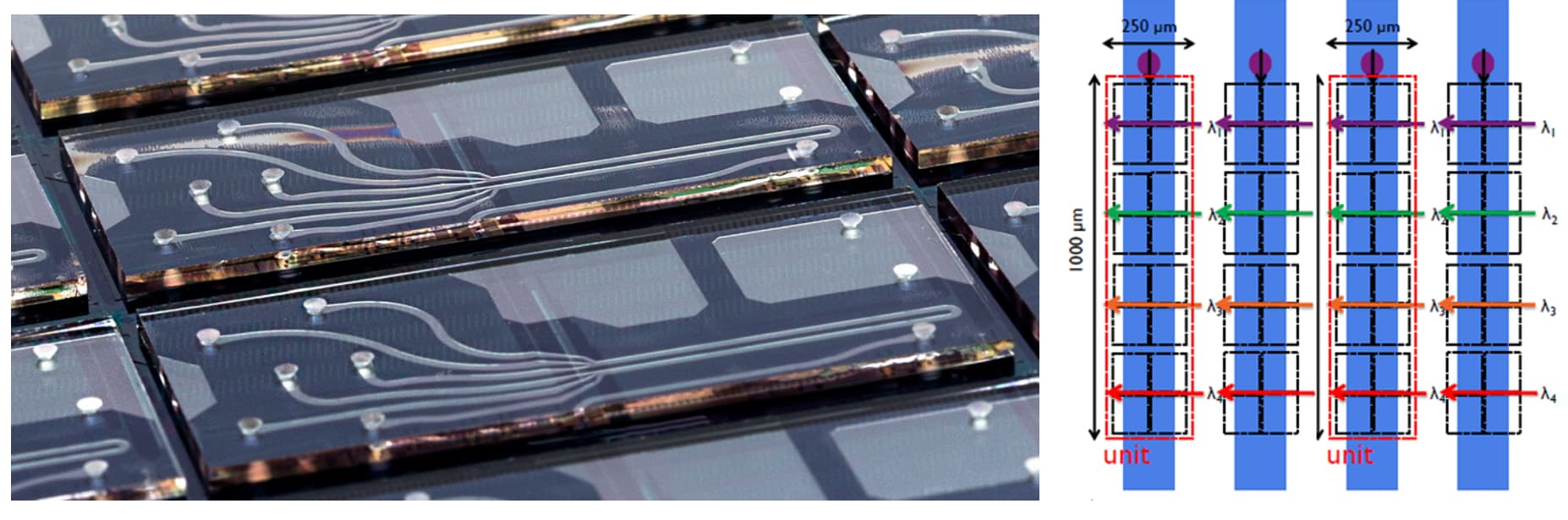
Initial concept of multimarker / color (4 waveguides = 4 markers can be detected simultaneously) cell sorter channels (4 in parallel). The 4 excitation wavelengths/colors can come from one laser or from multiple lasers.
Conclusion & future work
Although very promising results were achieved and innovative optimizations were developed to the cell sorter platform, further tests and developments are needed to integrate all these features into a true high-throughput, multi-marker cell sorter. There is still a long road to bring the demonstrated ideas to product maturity. This can only be achieved by working together with interested parties within e.g. the cell therapy scene and we are actively working towards such partnership.
Liesbet Lagae: “The jet flow cell sorter again is a great example of how semiconductor technology could revolutionize the diagnostics and therapeutics market. Thanks to the unique integration, parallelization and low-cost capabilities of semiconductor technology and the know-how of medical companies, we can change the life of many patients in the future.”
Want to know more?
- On the ERC website you can find this nice story of Liesbet Lagae’s projects, SCALPEL and Jetcell.
- If you would like to receive imec’s white paper on cell therapy, use this download link.
- Please contact us if you are interested in the paper “Micro vapor bubble jet flow for safe and high-rate fluorescence-activated cell sorting”. Other manuscripts are in preparation. Let us know if you want are interested in receiving them in the future.
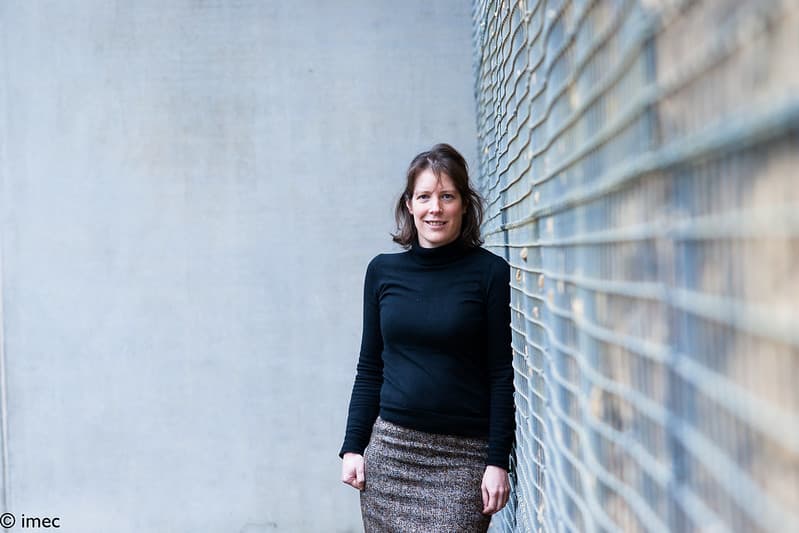
Liesbet Lagae is co-founder and Program Director of the Life Science Technologies in imec. In this role, she oversees the emerging R&D, the public funded activities and early business creation. She holds a PhD degree from the KU Leuven, Belgium for her work on Magnetic Random Access Memories. As a young group leader, she has initiated the field of molecular and cellular biochips leveraging silicon technologies at imec, Belgium. The life science program has grown from emerging activities to a mature business line that provides smart silicon chip solutions to the life science industry. Applications include medical diagnostics, point-of-care solutions, DNA sequencing, cytometry, bioreactors, neuroprobes, implants. She holds a prestigious ERC consolidator grant for developing a platform on single cell analysis and sorting. She has (co-) authored 125 peer-reviewed papers in international journals and holds 15 patents in the field. She is also part-time professor in nanobiotechnology at KU Leuven/Physics department.
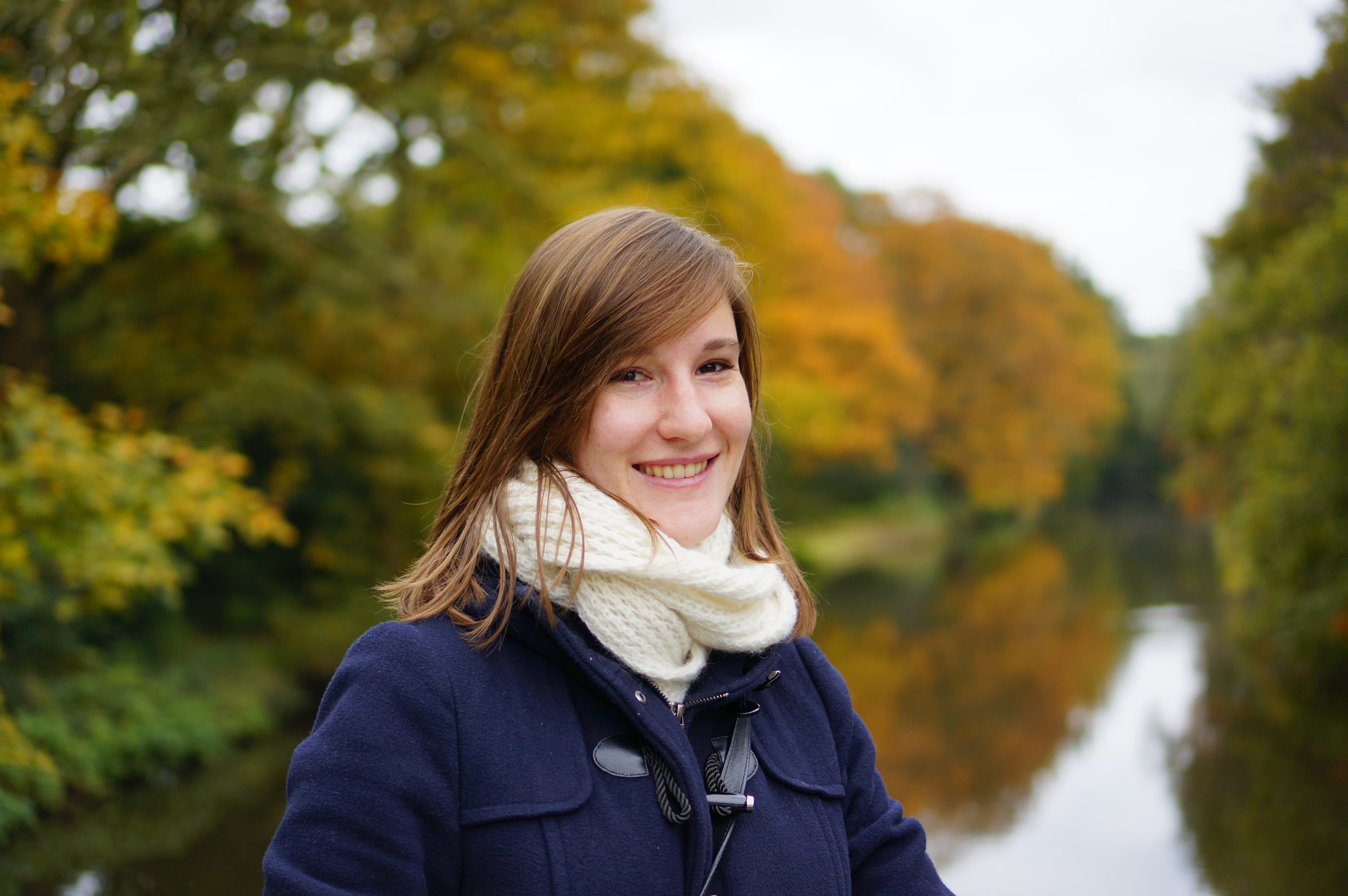
Sarah Libbrecht studied Biochemistry at the KU Leuven. In 2017 she obtained a PhD in biomedical sciences at the KU Leuven for her work on unravelling the role of the anterior olfactory nucleus in the adult neurogenesis of rodents using new neuromodulation techniques. In this study, she collaborated with imec on one of the first neuroprobes that combined stimulation and registration of neuronal activity. After obtaining her doctoral degree, she joined imec and worked as a senior bio-application specialist on the development of the cell sorter chip for cell therapy applications.
Published on:
2 October 2019