Source/drain contact resistance: a bottleneck for advanced Si CMOS technology
Source/drain contacts can be considered as the electrodes of the transistor, introducing and removing carriers to and from the transistor’s conduction channels. In traditional CMOS technology, metal-semiconductor (MS) contact schemes, with transition-metal silicides (such as nickel (Ni) silicides) on the metal side, are used to form the source/drain contacts. One popular scheme to fabricate these contacts makes use of the self-aligned silicide process, called SALICIDE – forming silicides that cover the entire source/drain surface.
But with shrinking transistor dimensions, the contact resistance at the source/drain – being reversely proportional to the contact area – increases accordingly. Hao Yu:
This problem of increasing contact resistance became dramatically worse from the 14nm node onwards, making the source/drain contact resistance the dominant parasitic resistance – and hence a major performance killer for advanced Si CMOS technologies. This fast increasing parasitic resistance urged us to develop improved source/drain contact schemes.
The contact resistance is like a ‘natural’ interface resistance that occurs whenever there is a contact between two different materials. “In general, two guidelines can be used to improve the contact resistance of metal-semiconductor contacts”, adds Hao Yu. “One is to increase the doping level at the semiconductor side, another is to optimize the interface quality between the metal and semiconductor.”
Ultralow contact resistivity: the new criterium for silicide candidates
Further investigations revealed that the metal-semiconductor contact resistivity (ρc) can be considered the main contributor to the source/drain resistance for small contact areas. Consequently, for future technology nodes, source/drain contacts with ultralow contact resistivity (i.e. below 2x10-9Ωcm2) are required to suppress parasitic resistance and ensure high transistor performance. “The contact resistivity rises due to discontinuous energy bands between the metal and the semiconductor”, explains Hao Yu. “A contact with an ultralow contact resistivity permits a high flux of carriers to transmit across the metal/semiconductor interface at a high rate.”
Although traditional Ni silicides provide low contact resistivities, fast diffusion of Ni in Si(Ge) limits its application in advanced nanoscale transistors. Hao Yu: “Therefore, the semiconductor industry encouraged imec to start looking for new contact schemes and new silicide materials, exhibiting ultralow contact resistivity and compatibility with CMOS processing.”
Schematic cross-sectional view of (a) a traditional transistor that utilizes SALICIDE techniques, and (b) a modern transistor. Note that silicides play no role in the lateral current distribution in the modern transistor.
The need for an adequate test vehicle
What was missing in industry, was a test vehicle that could accurately measure and evaluate structures with contact resistivities as low as 10-9Ωcm2. Hao Yu: “In the frame of my PhD study and with the help of the imec contact module team, I designed a unique planar test structure, based on a multiring circular transmission line model (MR-CTLM). The new test vehicle has a simple structure (with minimal process induced dimension errors), a large sampling capacity, and a high reproducibility – allowing an accurate characterization of ultralow contact resistivities.”
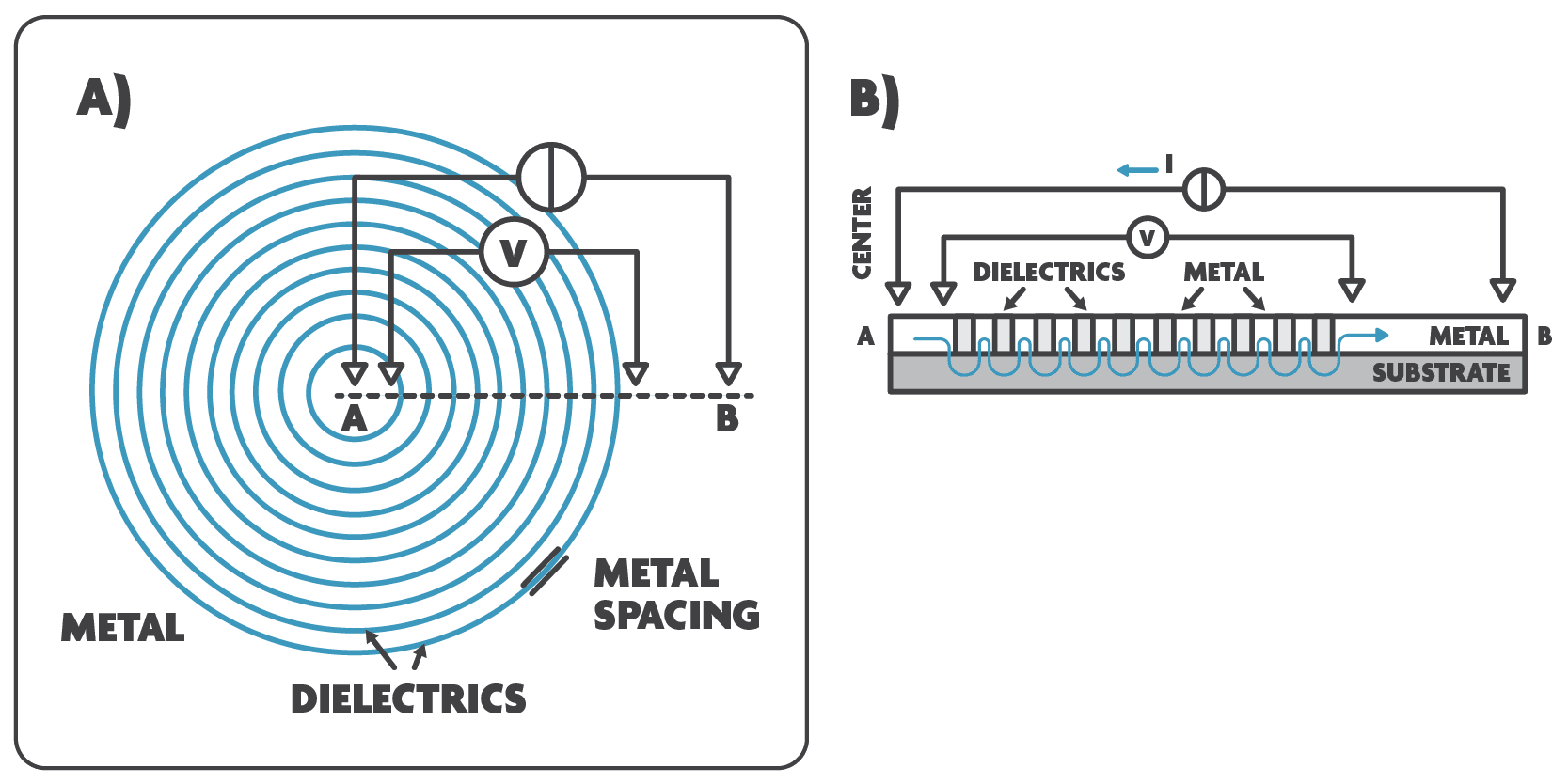
Schematics of the MR-CTLM test vehicle structure: (a) top view and (b) cross-sectional view.
Several major foundries have meanwhile accepted and implemented this MR-CTLM test structure. The structure was also reproduced by a number of universities. The MR-CTLM has enabled accurate investigations of ultralow contact resistivities that are close to the industry target. The simple fabrication of the MR-CTLM structure has also greatly facilitated and accelerated evaluation of various industry-relevant source/drain contact schemes.
Can metal-insulator-semiconductor contacts lead to low contact resistivities?
Several years ago, metal-insulator-semiconductor (MIS) source/drain contacts started to receive much attention. Hao Yu: “It was shown that inserting an ultrathin insulator between the metal and the semiconductor could help achieve a very low Schottky barrier height, and this should lead to low contact resistivities. As a first part of my PhD work, I evaluated this promising contact scheme in terms of contact resistivity and CMOS compatibility – for 7nm and beyond CMOS. I screened several insulator materials, including titanium (Ti)-dioxide. I found however two main drawbacks of the MIS source/drain contact approach, which prevented application of MIS contacts into advanced CMOS technologies. The problems are independent of the insulator candidate.”
One of the issues relates to the thermal stability of the MIS contacts, especially on n-type semiconductors. As a second issue, on the highly doped semiconductors that are relevant to the source/drain of the CMOS transistors, the Schottky barrier lowering benefit of the MIS contacts cannot compensate the insulator tunneling barrier induced blockage of carriers.
Novel titanium silicidation techniques
As a second part of his PhD work, Hao Yu switched to metal-semiconductor source/drain contacts, with Ti silicides on the metal side, and highly doped Si (n+) and SiGe (p+) on the semiconductor side.
After internal discussions and in close consultation with imec’s CMOS program partners, they developed three new techniques for titanium silicidation, with improved metal-semiconductor interfaces. With all three techniques, ultralow contact resistivities in the order of 10-9Ωcm2 could be achieved – as revealed by the proprietary MR-CTLM test vehicle.
The main difference between the techniques is the compatibility with state-of-the-art CMOS manufacturability. Hao Yu: “The conclusions we draw from the first technique allowed me to work out a second technique – which was better in terms of CMOS compatibility. The third came from the conclusions of the second one, and is most compatible with CMOS manufacturing. All three techniques were also evaluated by our partners.”
In a first technique, called pre-contact amorphization implantation, a Ge ion implantation step precedes the Ti deposition and contact formation. This step is known to improve the Ti-silicide nucleation and crystallinity. The lowest contact resistivity values were however achieved with silicides that were not fully crystalline, but in an amorphous state with embedded small Ti-silicide crystallites. Hao Yu: “The particular phase of low-ρc Ti silicides inspired me to develop a second technique, based on a TiSi co-deposition to form Ti silicides. With this technique, Ti-silicide crystallite formation can be enhanced. This technique also allowed us to improve the quality, reliability and reproducibility of the source/drain contacts.”
Cross-sectional TEM of an ultrathin co-deposited TiSi on SiGe ((a) before and (b) after 1 min at 450°C in N2).
Finally, a third technique was developed to answer the limitations of the TiSix deposition technique for compatibility with CMOS processing. “Conformal chemical vapor deposition (CVD)/atomic layer deposition (ALD) TiSix would be an optimal contact solution to the industry, but the technology is still under development. As a pioneering step, together with the industrial vendors, we explored the use of conformal deposition techniques such as ALD for depositing Ti”, adds Hao Yu. “This technique allowed us to create ALD Ti-based source/drain contacts, with ultralow ρc of 2x10-9Ωcm2.”
In the near future, Hao Yu will use the knowledge obtained in this PhD work for developing contact schemes for III/V-based devices, such as InGaAs or GaN – in the framework of imec’s high-speed analog/RF program for 5G mobile handsets.
Being a PhD student at imec: bringing ‘academic’ research closer to industry
At imec, PhD students have the excitement of working in an international environment with world-class expertise and using advanced equipment. Their research is key for imec’s research funnel.
Hao Yu: “I obtained my Master’s degree in semiconductor solid-state electronics at Fudan University, China. Afterwards, I got the chance to start my PhD at imec and KU Leuven. For me, one of the major advantages of working at imec is the close collaboration with industry. The problems I try to solve – although fundamental in nature – are among the real questions of the semiconductor industry. The feedback they give us makes me feel that my research matters, that it is important and relevant to industry.”
“In addition, to advance my research, I can largely rely on the expertise and knowledge shared by imec specialists in different fields”, adds Hao Yu. “The discussions I have with my supervisors (i.e., Marc Schaekers, my daily supervisor, and Nadine Collaert and Kristin De Meyer, my promotors), with some of the project managers and with members of other teams are extremely supportive. These discussions largely help us, PhD students, to grow faster and advance our research. I’m also very grateful to a number of professors at imec, who made me realize that, as a PhD student, I should always take the scientific value from my investigations.” The training opportunities offered by imec academy also largely contributed to his work and helped him broadening his horizon.
After five years at imec, Hao Yu can present an impressive list of publications. For this reason, he received the imec PhD student excellence award in 2018. He received other grants and prizes, including the prestigious IEEE electron device society PhD student fellowship award.
In 2019, Hao Yu will defend his PhD thesis. After that, he wants to stay at imec, where he plans to broaden his research field towards semiconductor junctions and RF oriented devices.
Want to know more?
- More details on the work on Ti silicides can be found in the paper ‘Titanium (Germano-)silicides featuring 10-9Ωcm2 contact resistivity and improved compatibility to advanced CMOS technology’, by Hao Yu et al. This paper was an invited contribution at the 2018 International Workshop of Junction Technology, and can be requested via this link.
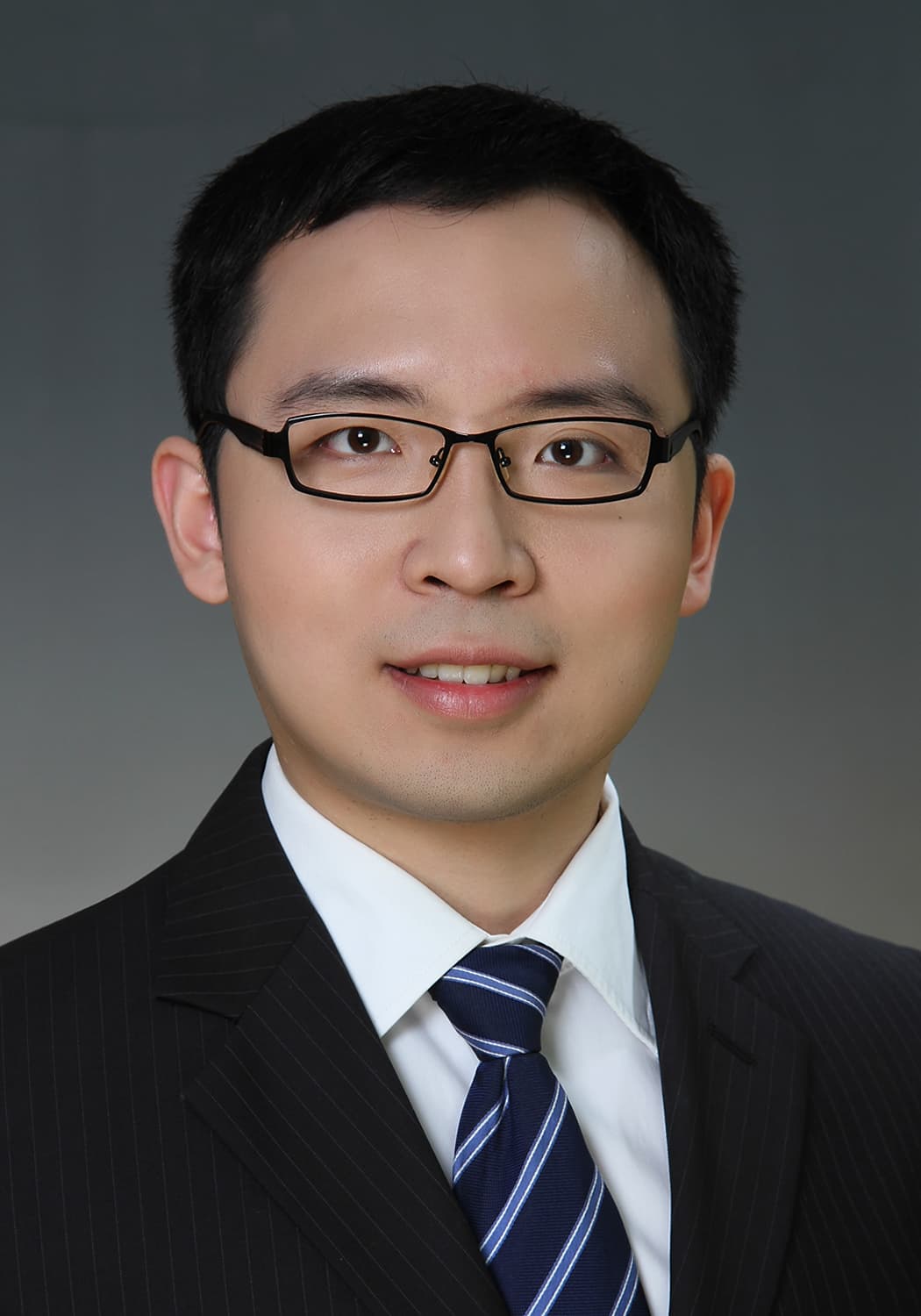
Hao Yu was born in Jinan, China. He received his B.S. and M.S. degrees in Microelectronics and Solid-State Electronics from Fudan University in 2010 and 2013, respectively. He received the China national graduate student scholarship award and Shanghai excellent graduate student award in 2013. Hao Yu is now a PhD candidate affiliated to imec and Katholieke Universiteit Leuven under supervision of Dr. Marc Schaekers, Dr. Nadine Collaert and Prof. Kristin De Meyer. He has been working on metal-semiconductor contact resistances and semiconductor junction characterizations. As high contact resistances become major obstacles that compromise performance of modern CMOS devices, Hao Yu has developed high-accuracy test vehicles and reported multiple contact schemes meeting the 10–9Ωcm2-contact-resistivity industrial target. He has authored and co-authored 17 articles to peer reviewed journals and 16 to international conferences. He received the IEEE electron device society PhD student fellowship award in 2017, the imec PhD student excellence award in 2018, and the China scholarship council award for outstanding self-financed students abroad in 2018. Since September 2018, Hao Yu has joined Dr. Niamh Waldron’s team at imec as a researcher working on IIIV-on-Si RF technologies.
Published on:
29 March 2019